This experiment uses inquiry and modeling techniques to develop an understanding of why transition metal aqueous solutions are colorful using d-orbital splitting when ligands and transition metal ions form complex ions. Students must have learned basic quantum theory especially understanding that absorbing light corresponds to a change in motion of the electron where the electron moves to an excited state of higher energy and when light is emitted the electron changes to a lower energy state. It may be helpful, but not necessary for them to have done calculations of light energy, frequency and wavelength as well as be familiar with the electromagnetic spectrum.
Day 1
The students are instructed on how to utilize the Vernier Spectrovis Plus equipment. They are briefed on how to fill cuvettes to about ¾ full, to hold the cuvettes on the ridged side so as not to leave smudges, how to calibrate the spectrophotometer and the correct settings. The data they are instructed to collect is the principal color or colors that are absorbed and which colors are transmitted. The chemicals used were Co(NO3)2, FeCl3, K4Fe(CN)6, Ni(C2H3O2)2, Cu(C2H3O2)2 and KMnO4. The anions used are not important, but it is helpful to have at least two with the same anion to eliminate the possibility of the color being dictated by the anion. A small amount of chemical is needed for the entire class and can easily be reused from year to year if put into containers that can be sealed. The solutions used were 0.2 M because that is what we had already made for previous labs. The KMnO4 solution was diluted to an unknown concentration so the absorbance was more reasonable. The concentration should not be too high or the absorbance will be beyond the measuring apparatus.
Figure 1: The experimental setup used for colorful salts
Students then ran all of the solutions and compiled their data into whiteboards.
Figure 2: Student data from the colorful salts experiment
Discussion
At this point the discussion began analyzing the data collected. There was not sufficient time for the entire discussion in the first period, but that worked out very well. There were two keys that I wanted to establish from the experiment and a cliffhanger for the following lesson. The first discussion point was that when a solution is (any color) it will transmit _____ and absorb ______. We first try and build an understanding of what we are seeing. If a solution is red, that means that the solution transmits red light and absorbs complementary colors such as green or blue. The second key for day 1 is to begin analyzing what each solution has in common so that we can develop a model to explain what is happening. In our first class of this we came up with the fact that the six solutions have the following in common (ionic, transition metal, metals and nonmetals are present in all six solutions, all metals are from period 4). This is a good time to briefly mention that the color must be from the cations since some anions are the same and the colors differ. The final component of day 1 is to preview that the next lesson will involve constructing a model to explain what is happening that makes these solutions colorful in the way that they are. We would like to use their commonalities to provide an explanation of the observed phenomena.
Day 2
Discussion
Day 2 began with our list of commonalities of our six solutions. I started class by saying that our list was insufficient and we must add to it. We then added that each solution was aqueous and that they were all colorful. At this juncture it was now time to begin developing a model. I brought out a solution of zinc nitrate to show that it was colorless and I also informed students that Sc(NO3)3 is also colorless. This is a good time for students to discuss in their lab groups what is needed for the solutions to be colorless. Someone should conclude that a partially filled set of d-orbitals is required. This is a key point and should be written down. It should also start the next question. The students are aware that in order to absorb light, an electron must move from one state (orbital) to another. Where are the 3d electrons moving to? And why can’t the 3d shell be full or empty? Now draw a set of 5 orbitals on the board to highlight this. At this point I reminded students that there are shapes of the d-orbitals and showed an image of them.
Figure 3: 3d orbitals from wikipedia
The images are best if they show axes and you can point out that some are along the axes (2) and some are between them (3). These orbitals all have the same energy, but will be influenced differently by the negative charge of ligands when forming a complex ion. Since all 6 solutions are aqueous, the water molecules have the oxygen end surrounding the transition metal ions. These water molecules are going to cause the d-orbitals to go from being 5 degenerate states to splitting into two groups. This splitting is what is key for the colorful transition metal cations.
Figure 4: d-orbital splitting from wikipedia
Now is a good time in the discussion to revisit Zn2+ being colorless and Sc3+ being colorless. The zinc ions have the electron configuration of 3d10. This will fill all 5 orbitals even when split and thus no transitions can occur from the bottom 3 to the top 2. For Sc3+ the electron configuration has no electrons in the d-shell and thus there are no electrons to shift from the bottom to the top. It is only when the d-shell is partially filled that the splitting causes colors of light to be absorbed.
Figure 5: Summary board at the end of our discussion
Figure 6: Whiteboard after going through zinc ions and scandium (III) ions being colorless
At this point we organize our model. When transition metal ions with partially filled d-orbitals are surrounded by a ligand (with negative charge)
- The d-orbitals split into different energies based on the proximity of the ligands
- The electrons can move between these new split levels absorbing visible light
- The color of the resulting solution is the light being transmitted
- The color is the complement of the light being absorbed
- This does not happen for Sc3+ or Zn2+ since the d-orbitals are either empty or completely full
To test our new model, we can then substitute other ligands in place of water to show that ligands with different amounts of negative charge and different proximity will cause a variety in the splitting of energy. Thus color will change when copper (II) ions have excess ammonia or chloride added.
If you would like to do this activity but do not have the Vernier equipment, you are welcome to utilize the video of the experiment and I also am placing photos of the absorbance curves below.
Figure 7: Red solution Co(NO3)2 absorbs blue and green light
Figure 8: Orange solution (FeCl3) ab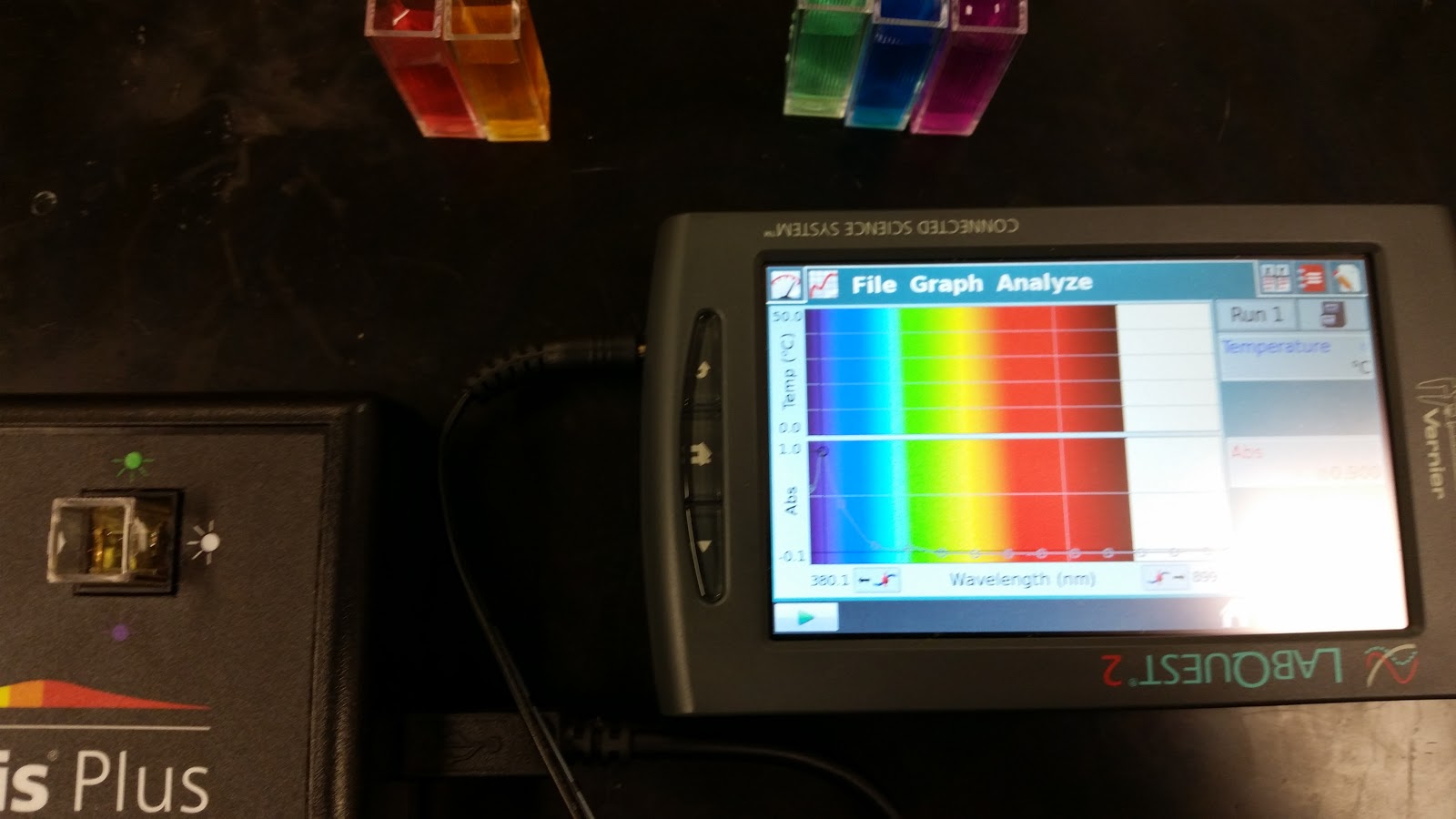
Figure 9: Yellow solution (K4Fe(CN)6) absorbs violet and blue light
Figure 10: Green solution (Ni(C2H3O2)2) absorbs red and violet light
Figure 11: Blue solution (Cu(C2H3O2)2) absorbs red and orange light
Figure 12: Violet solution (KMnO4) absorbs green and yellow light
Additional explanation for students can be found here:
Great enquiry setup for this topic. Thanks for sharing
ReplyDelete